Genome engineering and genome editing
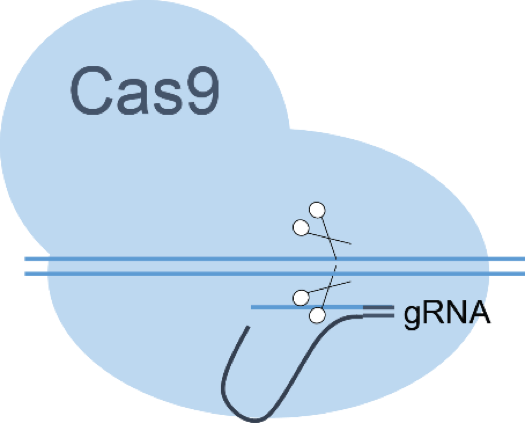
High-performing producer strains are essential for the biotechnological production of fine, specialty, and commodity chemicals. A suitable prokaryotic producer organism must not only be capable of overproduction of the compound of interest, but it also needs a stable genotype. This is usually tantamount to placing genetic information on the bacterial chromosome and most often requires methods that allow rapidly modification of multiple genomic functions. We are mainly using MAGE-based genome editing with CRISPR/Cas9 counter-selection. Applied examples are RBS optimization and translational or full gene knockouts for metabolic engineering, internal genomic tagging for protein knockdowns, and genomic integration of fluorescent proteins for biomass markers.
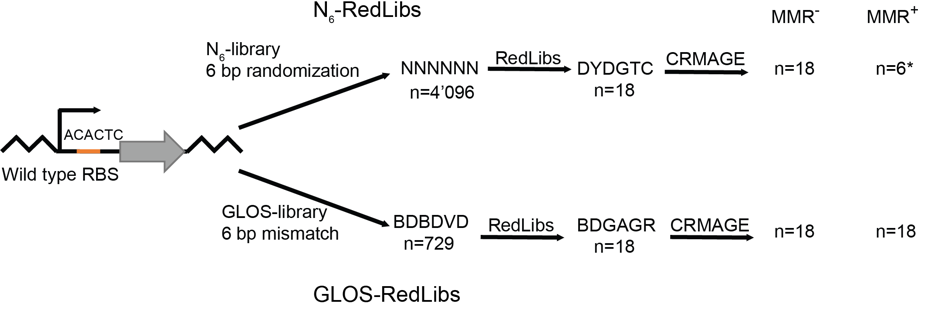
CRISPR/Cas9 counter-selection methods have become an important tool for prokaryotic genome engineering. In short, wild type cells are modified with oligonucleotides and λ-Red-based recombineering methods, resulting in up to 25% mutants in the population. To eliminate the “non-edited” cells, CRISPR/Cas9 counter-selection is applied. A guide RNA (gRNA) complementary to the wild type sequence recruits the Cas9 nuclease to the corresponding site on the chromosome, where it introduces a double strand break, resulting in cell death. However, the gRNA cannot anneal to the mutant cells and therefore these cells survive the CRISPR/Cas9 treatment, leading to an enrichment of the engineered mutant in the population.
We developed a method, called genome-library-optimized-sequences (GLOS), that can efficiently combine genome editing with RBS library approaches (RedLibs) in mismatch repair proficient strains.
References
S. Oesterle, D. Gerngross, S. Schmitt, T.M. Roberts and S. Panke: Efficient engineering of chromosomal ribosome binding site libraries in mismatch repair proficient Escherichia coli, Scientific Reports, 2017, external page DOI.
M. Jeschek, D. Gerngross and S. Panke: Rationally reduced libraries for combinatorial pathway optimization minimizing experimental effort, Nature Communications, 2016, external page DOI.
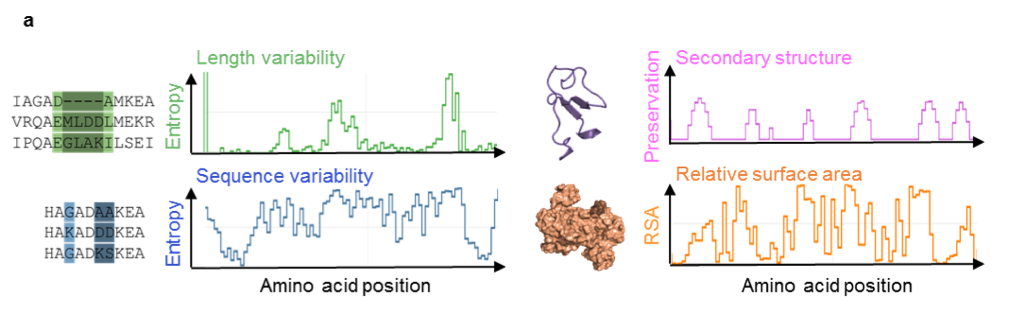
To design “switchable proteins” that can be hydrolyzed by site-specific proteases at the in vitro stage, we developed GapMiner to identify internal tagging sites in proteins, which can directly be inserted in the genome. To predict taggability of potential insertion sites, we chose three features: sequence and length variability, secondary structure and relative surface accessibility. With GapMiner, we can accelerate internal tagging site design and reduce the number of sites which need to be experimentally tested.
References
S. Oesterle, T.M. Roberts, L.A. Widmer, H. Mustafa, S. Panke and S. Billerbeck: Sequence-based prediction of permissive stretches for internal protein tagging and knockdown, BMC Biology, 2017, external page DOI.